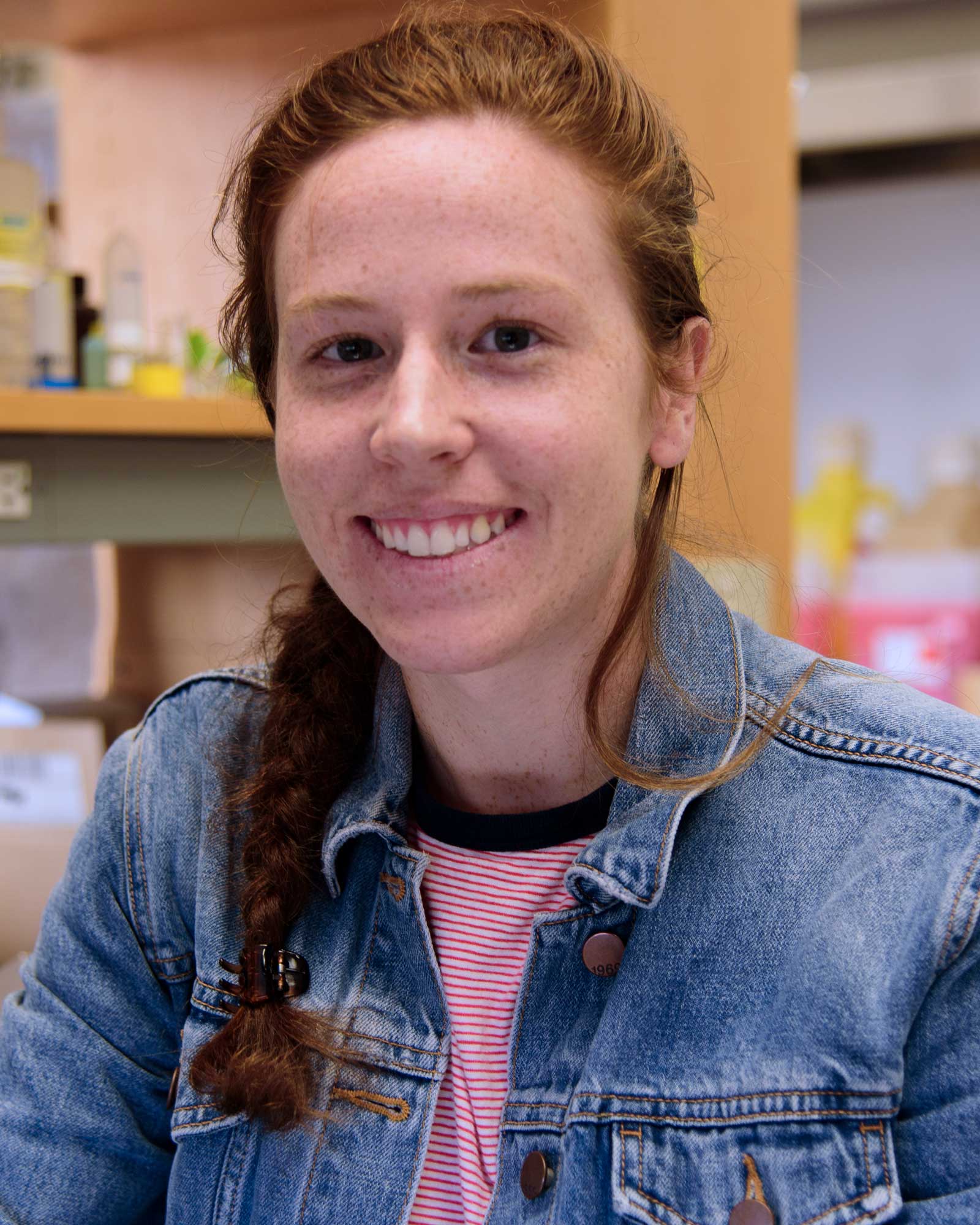
Maddie Pont
Alzheimer’s disease (AD) is the most common form of dementia, affecting over five million Americans today. There are currently no approved drugs which are shown to effectively modify the progression of the disease. Hundreds of clinical trials attempting drug intervention have failed over the past decade, a testament to the complexity of AD pathology. In the absence of effective treatment, the number of Americans suffering from the disease is expected to rise to around 16 million by 2050.
In the amyloid cascade hypothesis, soluble amyloid-beta (Aβ) spontaneously aggregates into highly β-sheet-rich oligomers and fibrils. Fibrils associate and precipitate as amyloid plaque on brain tissue and within blood vessel walls. Soluble oligomers and fibrils are thought to be neurotoxic, initiating a series of events leading to formation of neurofibrillary tangles, loss of synapse activity, and neuronal cell death. Intervention strategies are currently focused on reducing Aβ generation, enhancing Aβ clearance, or binding soluble Aβ to prevent aggregation.
Cystatin C (CysC) is a 13.4 kDa protease inhibitor which is highly secreted into the cerebrospinal fluid (CSF). CysC performs many housekeeping functions within the CSF, including regulating tissue degradation and cell death. CysC has also been reported to bind Aβ and inhibit aggregation. In vivo studies have found that CysC not only co-deposits with Aβ in AD plaques, but polymorphisms in the CST3 gene encoding CysC are correlated to risk of developing late-onset AD. In vitro binding studies between CysC and Aβ lack mechanistic detail, and little has been reported about the nature of this interaction.
In addition to its anti-amyloidogenic role in binding Aβ, a specific mutation of CysC (L68Q) is known to cause Hereditary Cystatin C Amyloid Angiopathy (HCCAA), another amyloid disease affecting the vasculature of the central nervous system. Fibrillation was proposed by others to be a consequence of CysC dimerization via a domain-swapping mechanism. We hypothesized that CysC amyloid propensity is related to its strong interaction with Aβ. We investigated the CysC fibril-forming mechanism in order to further understand this interaction.
We first developed a protocol for producing and purifying recombinant human CysC in E. coli, using an intein-based affinity tag for ease of purification. The self-cleavable chemistry of the intein tag allowed a simple, one-step preparation procedure to isolate full-length CysC. Upon purification, we found CysC was a mixture of monomers and oligomers. We separated and further characterized the oligomers. The oligomers were not domain-swapped, which is the first reported instance of oligomerization without domain-swapping in CysC. We also found that non-swapped oligomeric CysC had pre-amyloid characteristics and inhibited Aβ aggregation much more potently than monomeric CysC.
We next investigated the CysC fibrillation mechanism. The propagated domain-swapping (PDS) hypothesis, wherein CysC associates into fibrils via an open-ended domain-swapping fashion, has been used to explain CysC fibrillation for nearly 20 years. We produced a single-point mutant of CysC, V57N, that retains native folded structure but does not undergo domain-swapping. V57N formed fibrils faster than wild-type, contradicting the PDS hypothesis. Furthermore, non-swapped oligomers were resistant to fibril formation. We conclude that CysC fibrillation likely initiates from the monomeric state and is related to the stability of the native protein fold, and does not fibrillize via propagated domain-swapping.
CysC and Aβ interaction in vivo is likely modified by other proteins, notably cathepsin B (CatB). CatB is a cysteine-type protease typically found in acidic cellular compartments, but which can be transferred to the CSF through secretory pathways or by apoptosis. Because CatB retains some of its activity in the neutral pH of CSF, CysC has the important job of specifically and potently inhibiting leaked CatB. CatB is known to degrade soluble Aβ into non-aggregating fragments, forming a natural Aβ-clearance mechanism.
We modeled the CysC/CatB/Aβ interaction by considering binding, degradation, and aggregation reactions. We performed in vitro experiments to measure critical equilibrium and kinetic rate constants. We found that our model could accurately predict the aggregation behavior of the ternary system in vitro. We developed a dimensionless parameter to describe the ratio of characteristic degradation and aggregation timescales, and found that the steady-state model solution was controlled by this dimensionless parameter.
Researchers studying disease-modifying therapies in animal models can easily calculate this dimensionless parameter and predict aggregation behavior, simply by knowledge of typical CysC/CatB/Aβ levels in the model. This model can be extended to consider more protein interactions relevant to AD pathology. Additionally, it can be adapted to include an Aβ generation step, in which CatB is also known to play a role.